Michael E. Dailey, Ph.D.
B.S. (Biology), Geneva College
Ph.D. Biology & Biomedical Sciences (Neural Science), Washington Univ. (St. Louis)
Post-doc, Molecular & Cellular Physiology, Stanford Univ. Medical School (with Stephen J Smith)
My Contributions to Science
[Google Scholar citations for each article as of 1/1/2016]
1. Cytoarchitectural organization of neuronal growth cones
Since the time of Ramon y Cajal it has been thought that the specialized tip of a growing axon, called the “growth cone,” plays a critical role in the elongation and guidance of neuronal processes, yet the mechanisms underlying growth cone function were not understood. My work as a graduate student with Paul Bridgman (Washington Univ. – St. Louis) in the late 1980s helped provide a structural framework for understanding how the cytoskeleton and membrane organelles within growth cones are organized and remodeled over time to enable axonal growth and guidance. The fine structure of growth cones was studied using whole-mount intermediate-voltage electron microscopy of rapidly-frozen sympathetic neurons in culturea,c,d, and time-lapse imaging of fluorescently labeled membrane organelles was performed to study the dynamic remodeling of membrane organelles during axon extensionb,d. These studies identified movements and interactions of cytoplasmic elements that regulate both the advance of membrane organelles into growth cones as well as membrane traffic between intracellular membrane pools and the plasmalemma. This information, incorporating both ultrastructure and dynamic remodeling of organelles, helped lay a foundation for current models of growth cone organization and function underlying growth cone steering and directional axon outgrowth.
a. Bridgman PC, Dailey ME (1989) The organization of myosin and actin in rapid frozen nerve growth cones. J. Cell Biol. 108:95-109. PMID: 2642912. [>190 citations]
b. Dailey ME, Bridgman PC (1989) Dynamics of the endoplasmic reticulum and other membranous organelles in growth cones of cultured neurons. J. Neurosci. 9(6):1897-1909. [journal cover] PMID: 2723756. [>60 citations]
c. Dailey ME, Bridgman PC (1991) Structure and organization of membrane organelles along distal microtubule segments in growth cones. J. Neurosci. Res. 30:242-258. PMID: 1795407. [>30 citations]
d. Dailey ME, Bridgman PC (1993) Vacuole dynamics in growth cones: correlated EM and video observations. J. Neurosci. 13(8):3375-3393. PMID: 8340814. [>45 citations]
2. Imaging the dynamics of neuronal migration, axon and dendrite outgrowth, and synapse formation in mammalian brain tissues
The formation of appropriate neural circuits involves the proper positioning of neurons as well as the outgrowth and interaction of axons and dendrites in target regions. As a postdoc with Stephen Smith (Stanford) in the 1990s, I helped pioneer time-lapse confocal imaging approaches to visualize neuronal migrationa as well as outgrowth of axons and dendritesb,c in live developing mammalian brain tissue slices. We were the first to directly demonstrate that target dendrites are highly dynamic structures and contribute to the formation of synaptic connections via filopodia that actively initiate contact with axons in developing brain tissuesc. After establishing my own lab at the University of Iowa in 1996, I set out to directly visualize the formation of postsynaptic structures by imaging transfected neurons expressing GFP-tagged postsynaptic proteins (e.g., PSD95 and CaMKIIα). My team showed that developing postsynaptic densities within dendrite shafts, spines, and spine precursors are highly dynamic structures, permitting rapid formation and remodeling of new synaptic connections in developing CNS tissuesd. These studies provided the first real-time visualization of synapse formation and remodeling in live mammalian brain tissues. Subsequently, we identified factors that regulate the assembly and remodeling of postsynaptic scaffold proteins during development and following experimentally-induced epileptiform activitye.
a. O'Rourke NA, Dailey ME, Smith SJ, and McConnell SK (1992) Diverse migratory pathways in the developing cerebral cortex. Science 258:299-302. [journal cover] PMID: 1411527. [>350 citations]
b. Dailey ME, Buchanan J, Bergles D, Smith SJ (1994) Mossy fiber growth and synaptogenesis in rat hippocampal slices in vitro. J. Neurosci. 14(3):1060-1078. PMID: 8120613. [>100 citations]
c. Dailey ME, Smith SJ (1996) The dynamics of dendritic structure in developing hippocampal slices. J. Neurosci. 16(9): 2983-2994. [journal cover] PMID: 8622128. [>500 citations]
d. Marrs G, Green SH, Dailey ME (2001) Rapid formation and remodeling of postsynaptic densities in developing dendrites. Nature Neurosci. 4(10):1006-1013. PMID: 11574832. [see also: News & Views, pp. 967-968] [>250 citations]
“Recommended” by F1000Prime
e. Ahmed R, Zha XM, Green SH, Dailey ME. (2006) Synaptic activity and F-actin coordinately regulate CaMKIIalpha localization to dendritic postsynaptic sites in developing hippocampal slices. Mol. Cell. Neurosci. 31(1):37-51. PMID: 16214364. [>20 citations] “Recommended” by F1000Prime
3. A new approach to study astrocyte structure, development, and remodeling
Astrocytes are among the most numerous cells in the mammalian brain and are now understood to be critical functional neural elements, yet studies of astrocyte structure–function relationships had been hampered by the lack of an in vitro experimental system that promotes normal astrocyte development in the context of other neurons and glial cells in a neuropil-like tissue organization. We pioneered an in vitro approach to study the functional architecture, development, and dynamic remodeling of astrocytes in live mammalian brain tissues using ballistic labeling in organotypic hippocampal tissue slice cultures. Using a novel membrane-targeted GFP (LCK-GFP), we demonstrated that astrocytes in slice cultures can retain a highly complex 3D morphology with many fine spine- or veil-like protrusions that are structurally dynamic and show localized calcium spikesa. Further, we used this system to study the subcellular distribution and dynamic remodeling of the predominant glutamate transporter GLT-1 (EAAT2) in developing astrocytes. We showed that GLT-1 is clustered in astrocyte membranes, and time-lapse imaging showed that GFP-GLT-1 clusters are remodeled on a timescale of minutesb. Moreover, using pharmacological manipulations we demonstrated that neuronal activity influences both the organization of glutamate transporters in developing astrocyte membranes and their position relative to neural synapses.
a. Benediktsson AM, Schachtele SJ, Green SH, Dailey ME (2005) Ballistic labeling and dynamic imaging of astrocytes in organotypic hippocampal slice cultures. J. Neurosci. Methods 141:41-53. PMID: 15585287. [>100 citations]
b. Benediktsson AM, Marrs GM, Tu JC, Worley PF, Rothstein JD, Bergles DE, Dailey ME (2012) Neuronal activity regulates glutamate transporter dynamics in developing astrocytes. Glia 60(2):175-188. PMID: 22052455. PMC3232333. [>50 citations]
Identified as a “Top 10 Original Article Published in the journal GLIA in 2012”
“Recommended” by F1000Prime
4. Regulation of microglial motility, migration, and phagocytosis in the developing CNS
My lab has been studying the functional behavior and regulation of microglial cells for nearly 20 years. We helped pioneer the use of time-lapse fluorescence confocal imaging to study microglia in acutely isolated and cultured rodent brain slices, which we used to define morphological and functional changes during early stages of microglial activation in live developing brain tissuesa. This work also identified microglial behaviors involved in the initial contacts and clearance of dead cells in brain tissuesb,f. We helped identify P2Y12R-based purinergic signaling mechanisms involved in triggering microglial activation and directing microglial homing to dead and dying brain cellsc,d. We are now studying these events in in vivo models of brain injury, including developmental alcohol exposuree and stroke (see below). (* denotes undergraduate student authors)
a. * Stence N, Waite M, Dailey ME (2001) Dynamics of microglial activation: A confocal time-lapse analysis in hippocampal slices. Glia 33:256-266. PMID: 11241743. [>300 citations]
b. * Petersen MA, Dailey ME (2004) Diverse microglial motility behaviors during clearance of dead cells in hippocampal slices. Glia 46:195-206. PMID: 15042586. [>100 citations]
F1000Prime “Must Read”
c. Haynes SE, Hollopeter G, Yang G, Kurpius D, Dailey ME, Gan W-B, Julius D (2006) The P2Y12 receptor regulates microglial activation by extracellular nucleotides. Nat. Neurosci. 9(12):1512-1519. PMID: 17115040. [>500 citations]
F1000Prime “Must Read”
d. Kurpius D, *Nolley EP, Dailey ME (2007) Purines induce directed migration and rapid homing of microglia to injured pyramidal neurons in developing hippocampus. Glia 55(8):873-884. [journal cover] PMID: 17405148. [>60 citations]
e. Ahlers KE, Karaçay B, Fuller L, Bonthius DJ, and Dailey ME (2015) Transient activation of microglia following acute alcohol exposure in developing mouse neocortex is primarily driven by BAX-dependent neurodegeneration. Glia 63(10):1694–1713. PMID: 25856413.
f. Eyo U, * Miner S, Weiner JA, and Dailey ME (2016) Developmental changes in microglial mobilization are independent of apoptosis in the neonatal mouse hippocampus. Brain, Behavior, and Immunity 55:49–59. PMID: 26576723.
5. Microglia and Stroke
We are investigating the effect of stroke on microglial survival and function, with the goal of understanding how microglia impact stroke sequelae. Research in perinatal stroke, a leading cause of lifelong disability, has implicated microglia as targets for therapeutic intervention during stroke. Although microglial responses are complex, some work in developing rodents suggests that microglia may limit brain damage after stroke. Thus, preserving microglia may provide a strategy for promoting tissue restoration after stroke. Using an in vitro model of stroke (oxygen-glucose deprivation, OGD) in acutely isolated hippocampal slices from neonatal mice, we demonstrated that microglia are vulnerable to both transient and sustained OGD, and many microglia die within hours after OGD onseta. Both in vitro and in vivo models are being employed to elucidate mechanisms regulating stroke-induced microglial cell death. Using pharmacological and genetic approaches in acutely-isolated brain slices and cultured BV2 cells, we showed that OGD causes Ca2+-dependent microglial cell death that is mediated in part by P2X7-type purinergic receptorsb. This was the first demonstration of a purinergic receptor regulating microglial survival in brain tissues. We are now collaborating with Dr. A. Chauhan (U. Iowa) to define microglia responses in a middle cerebral artery occlusion model of stroke in P2X7R null mice. We are also exploring preliminary evidence that adenosine protects against OGD-induced microglia cell death, with a goal of untangling the complex regulation of microglial biology by purines.
a. Eyo U, Dailey ME (2012) Effects of oxygen-glucose deprivation on microglial motility and viability in developing mouse hippocampal tissues. Glia 60(11):1747-1760. [journal cover] PMID: 22847985 [PMC3786781]. [>10 citations]
b. Eyo UB, *Miner SA, Ahlers KE, Wu L-J, Dailey ME (2013) P2X7 receptor activation regulates microglial cell death during oxygen-glucose deprivation. Neuropharmacology 73:311–319. PMID: 23770338. [PMC3786777]. [>15 citations]
6. Methodology
Imaging
- Terasaki M, and Dailey ME (1995) Confocal microscopy of living cells. In, Handbook of Biological Confocal Microscopy, 2nd Edition (J.B. Pawley, ed.) New York: Plenum, pp. 327-346. [>65 citations]
- Dailey ME, and Waite M (1999) Confocal imaging of microglial cell dynamics in hippocampal slice cultures. Methods 18(2):222-230. PMID: 10356354. [>70 citations]
- Dailey ME, Manders E, Soll D, and Terasaki M (2006) Confocal microscopy of living cells. In, Handbook of Biological Confocal Microscopy, 3rd Edition (J.B. Pawley, ed.) pp. 381-403. New York: Springer. [>35 citations]
- Dailey ME et al. “Introduction to Live-Cell Imaging Techniques.” 2 Aug 2006. http://www.microscopyu.com/articles/livecellimaging/index.html.
- Dailey ME, Marrs GS, Kurpius D (2011) Maintaining live cells and tissue slices in the imaging setup. Chapter 33 in, Imaging in Neuroscience: A Lab Manual. (Helmchen F, Konnerth A) Cold Spring Harb Protoc. Apr 1; PMID: 21460059.
- Dailey ME, Eyo U, Fuller L, Hass J, Kurpius D (2013) Imaging microglia in brain slices and slice cultures. Cold Spring Harbor Protocols. pdb. prot079483.
Slice cultures
- Fuller L, and Dailey ME (2007) Preparation of Rodent Hippocampal Slice Cultures. Cold Spring Harbor Protoc. Oct 1;2007:pdb.prot4848. PMID: 21356949. [>20 citations]
7. Review Articles
- Eyo U, Dailey ME (2013) Microglia: Key Elements in Neural Development, Plasticity, and Pathology. J. Neuroimmune Pharmacol. 8:494-509. PMID: 23354784. [>40 citations]
My Contributions to Teaching Science
Miakotina O, Dailey ME (2014) Neuroprogenitor Cell Differentiation in Culture. Pp. 256-269 in Tested Studies for Laboratory Teaching, Vol. 35 (K. McMahon, Editor). Proceedings of the 35th Conference of the Assoc. for Biology Laboratory Education (ABLE), 242 pages.
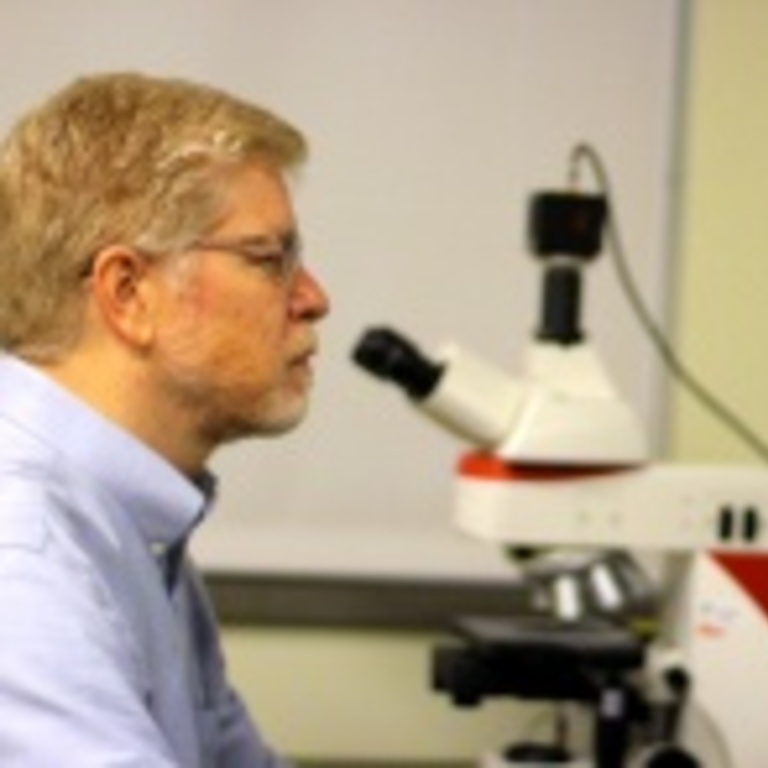